Ebola has a cousin — the highly lethal Marburg virus (MARV), which is closely related to the Ebola virus. Both are members of the Filoviridae family. MARV is highly infectious and causes a haemorrhagic fever in humans and primates, with mortality rates of over 80% in some outbreaks.
The World Health Organization (WHO) lists Marburg (and Ebola) virus in the top 10 on their list of diseases that pose the greatest public health risk due to their epidemic/pandemic potential and the lack of sufficient countermeasures.
While the obvious global infectious disease threats are bird flu, novel zoonotic and human influenza viruses, and severe acute respiratory syndrome (SARS) coronaviruses, many other threats cannot be ignored (see Table 1).
Chikungunya virus disease |
Crimean–Congo haemorrhagic fever |
Dengue fever |
Ebola/Marburg |
Influenza viruses: bird flu (H5N1); novel zoonotic and human viruses |
Japanese encephalitis virus |
Lassa fever |
Middle East respiratory syndrome coronavirus (MERS-CoV) |
Mpox |
Nipah and virus henipaviral diseases |
Oropouche virus |
Rift Valley fever |
Severe acute respiratory syndrome (SARS) and SARS-CoV-2 (COVID-19) |
Yellow fever |
Zika virus |
All are a great threat to travellers, workers and residents in endemic areas and have the potential to cause epidemics and pandemics.
There is also international concern regarding the potential rise of a new serious international epidemic caused by a pathogen not seen before, labelled as Disease X, which is likely to emerge due to a zoonosis with efficient transmission in humans. Crucially, environmental factors such as global warming or tropical deforestation may also play an important role.
The WHO reviews and updates its list of pandemic candidates and has developed research and development roadmaps enabling preparedness for each threat.
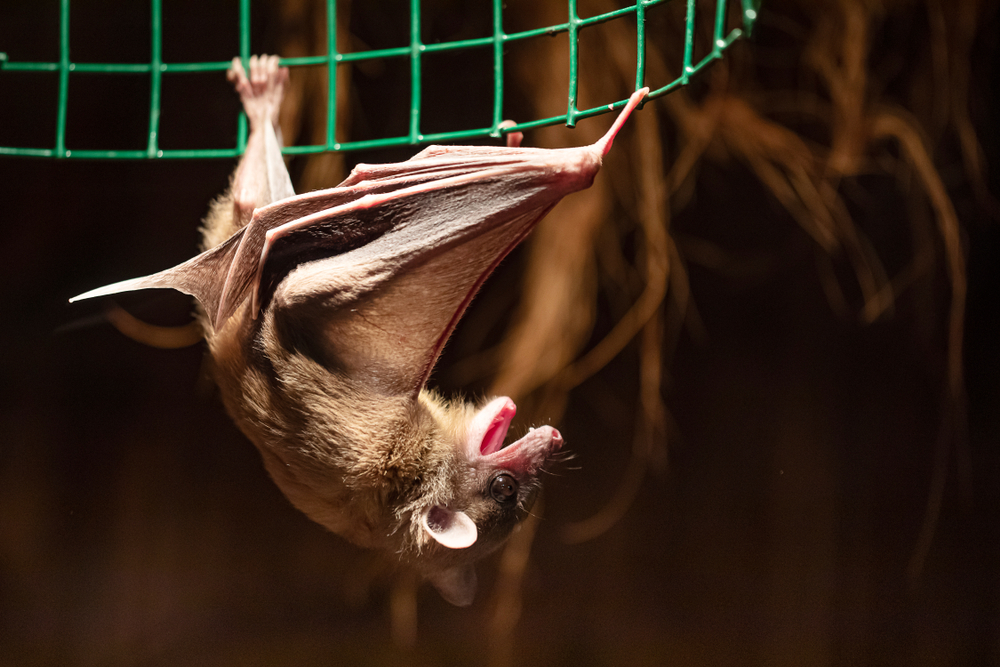
From laboratory animals to humans
MARV was first identified in 1967 in laboratory researchers working in Marburg, Frankfurt, and Belgrade, and was traced to African Green Monkeys, which were imported from Uganda for research purposes. However, the natural hosts and source of infection are various species of bats (Rousettus aegyptiacus, Hipposideros caffe). In Marburg, there were 37 cases and 9 deaths with some cases spreading through household or nosocomial contact.
Around Africa
To date, 705 cases of Marburg virus disease (MVD) have been reported globally, predominantly in Africa; in Uganda, the Democratic Republic of the Congo (DRC), Kenya, Angola, the United Republic of Tanzania, Equatorial Guinea, Ghana and now Rwanda. The two largest outbreaks have occurred in the DRC, from 1998 to 2000, with 154 cases, and in Angola, from 2004 to 2005 with 374 cases. Case fatality rates were 83% and 88% respectively. In October 2024, Rwanda reported its first outbreak of MVD, involving at least 62 cases to date and 15 deaths. Most of those infected in the outbreak were health care workers, particularly those in intensive care units, and hundreds of contacts are being followed up.
Outside of Africa, single cases were reported in travellers from Africa returning to the Netherlands (2008) and USA (2008) and there was also a case in Russia affecting a laboratory worker (1990).
Animal reservoirs
Egyptian Fruit Bats (Rousettus aegyptiacus) are distributed across Africa and are known to inhabit caves and mines, and are the natural reservoir for MARV. Studies have found evidence of MARV in bat populations, which shed the virus in saliva, urine and faeces but are asymptomatic. Non-human primates are also susceptible to MARV infection and severe disease, and serve as intermediaries between bats and humans, but are not reservoirs of infection.
Transmission to humans
Human-to-human transmission occurs via direct contact with the blood, secretions, organs or other bodily fluids of infected individuals, including deceased persons. Infected primates can transmit the virus to humans through direct contact and from humans eating bushmeat (meat that comes from wild animals). During the 1998–2000 outbreak in the DRC, most human cases were linked to contact with infected chimpanzees. Similarly, during the 2005 outbreak in Angola, exposure to infected primates likely contributed to the virus’s spread.
Health care settings are particularly vulnerable to spreading the virus if adequate infection control measures are not observed, such as during the 1998–2000 outbreak in the DRC. Health care workers can also become infected if personal protective equipment is unavailable or inadequate.
Human activities that increase contact with bats, such as mining or tourism in bat-inhabited mines and caves, and bushmeat hunting, facilitate the spillover of the virus from bats to humans. Miners working in deep caves often come into contact with bat secretions, increasing their risk of infection, presenting an occupational hazard to the industry.
Symptoms and pathogenesis
MVD presents similarly to Ebola virus disease, with an incubation period ranging from 2 to 21 days. Initial symptoms include fever, chills, headache and myalgia, which are often followed by gastrointestinal symptoms like nausea, vomiting, abdominal pain and diarrhoea. As the disease progresses, patients experience jaundice, multiorgan failure and shock.
MARV also causes extensive vascular damage, which leads to widespread haemorrhages, often culminating in death within 8 to 9 days of the onset of symptoms in severe cases.
MARV targets several cell types, including dendritic cells, macrophages and hepatocytes; infection results in a dysregulated immune response, leading to severe haemorrhagic symptoms, and a high level of pro-inflammatory cytokines (cytokine storm), which is responsible for systemic damage. The VP35 and VP24 proteins of MARV counteract host immune responses by blocking interferon (IFN)-α/β production and inhibiting signalling downstream of IFN-α/β and the IFN-γ receptors. VP35 also represses IFN gene transcription. It is clear that VP35 IFN-antagonist activity is critical for severe MVD.
Diagnosis of MVD can be challenging due to the overlap in early symptoms with other febrile illnesses such as malaria or typhoid fever. Laboratory confirmation typically involves polymerase chain reaction, or enzyme-linked immunosorbent assays to detect MARV antibodies, or electron microscopy to visualise the virus.
Are vaccines available?
There is no approved vaccine for MARV, although some progress has been made. The most promising vaccine candidate uses a live attenuated vesicular stomatitis virus as a vector to express the glycoprotein of the virus, inducing a robust immune response; however, this work has only been done using animal models. MVA-BN-Filo is another candidate vaccine and contains antigens of both Ebola and Marburg viruses, and showed protective effects to both in animal models. DNA-based vaccines and those using adenovirus vectors to express MARV glycoproteins also show promise.
What about therapies?
There is no approved antiviral treatment for MVD; however, several experimental therapies are in preclinical development or clinical trials and include the use of monoclonal antibodies, antiviral drugs, and interferon-based therapies. Symptomatic treatment of acute infection includes balancing fluid and electrolyte levels, maintaining oxygen level and blood pressure, replacing blood and clotting factors as necessary, and controlling the cytokine storm.
Monoclonal antibodies are among the most promising therapies for MVD as they can specifically target viral proteins to neutralise the virus. Monoclonal antibody cocktails, such as ZMapp (developed for Ebola) and MBP134, successfully treated non-human primates infected with MARV and were also shown to give protection after exposure to MARV.
Remdesivir, which was initially developed for Ebola virus disease, is a nucleotide analogue that inhibits RNA polymerase. It has broad-spectrum antiviral activity against filoviruses (including MARV). In non-human primate studies, remdesivir improved survival when administered after exposure to MARV. However, its effectiveness in humans is unknown.
The antiviral favipiravir was originally developed for influenza and inhibits viral replication by targeting the RNA-dependent RNA polymerase. Preclinical studies have shown it to be effective against filoviruses in animal models; however, like other therapies its efficacy in humans has not been established. The drug is currently undergoing trials for its potential use in treating a variety of viral haemorrhagic fevers. Synthetic interferons have been evaluated for their efficacy in treating filoviruses in non-human primates; however, their success in humans has been mixed.
Novel treatments have also been explored, including the use of herbs, plant metabolites, phytochemicals, immune-boosting nutritional foods and nutraceuticals, and have been used to treat rare viral diseases for which there is no treatment. Furthermore, there have been a number of observational studies targeting the host response to life-threatening infection causing excessive inflammation, by the use of inexpensive repurposed generic drugs such as statins, angiotensin-converting enzyme inhibitors, and angiotensin receptor blockers. Such approaches may help maintain or restore endothelial barrier integrity and need further exploration.
Prevention and control
MVD is lethal and poses a significant public health threat due to rapid human-to-human transmission and lack of therapeutic interventions.
Prevention and control efforts must focus on a variety of strategies including: enhanced surveillance in areas where Marburg virus is active; monitoring bat populations for viral presence; serological surveys in humans and animals confirming exposure; public health education on the dangers of consuming bushmeat, the handling of sick or dead animals, and the risk of entering bat-inhabited caves; improved health care infrastructure as currently affected areas have limited health care resources; effective vaccines to Marburg virus and their efficient delivery to those at risk when available; and, ensuring that antivirals and other drugs are available to health care infrastructures in affected areas.
Specific efforts can also be made to raise awareness among both miners and tourists/travellers, which are in the highest risk categories. For miners, the use of personal protective equipment, such as gloves and masks, will reduce the risk of exposure. Tourist and travellers should avoid bat-inhabited caves; access to such areas should be restricted with clear signage and protective barriers.
Environmental factors
Ecological changes, such as deforestation and urbanisation, may also influence the dynamics of virus transmission. For example, deforestation can displace bat populations from their natural habitats and allow closer contact with humans, increasing the likelihood of zoonotic spillover events. Moreover, climate change may play a role in altering the distribution of bat species, potentially expanding the range of zoonotic Marburg virus transmission. Changes in rainfall patterns and temperature will likely influence the availability of food for bats, leading them to migrate to new areas. Mitigating the impact of these changes requires ongoing surveillance as well as research at the animal–human interface.
A recent good example of the impact of ecological changes on virus epidemiology is the Oropouche virus, an arbovirus transmitted by midges and some mosquitoes, which has, until recently, been restricted to South America, especially the Amazon Basin. However, in June 2024, Cuba reported its first confirmed Oropouche cases where the virus has now become endemic and a few cases were also reported in Italy and Spain, in travellers returning from Cuba. Deforestation and ecological changes are thought to be factors in the sudden spread of this virus.
Greater efforts are needed to control and understand the emergence of zoonotic viruses through enhanced surveillance, targeted research, awareness of environmental factors at the animal human interface and the timely development of effective diagnostics, vaccines and therapeutics.
Professor Booy is a clinical epidemiologist and an honorary professor at the University of Sydney.
Professor Gary Grohmann is a consultant virologist and adjunct professor at the University of Sydney.
The statements or opinions expressed in this article reflect the views of the authors and do not necessarily represent the official policy of the AMA, the MJA or InSight+ unless so stated.
Subscribe to the free InSight+ weekly newsletter here. It is available to all readers, not just registered medical practitioners.
If you would like to submit an article for consideration, send a Word version to mjainsight-editor@ampco.com.au.
The reason an African virus (aka ‘Green Monkey Fever’) is called ‘Marburg’ after a German city, is because clever researchers decided that transporting it transcontinentally was a wise move.
A similar spreading event occurred with Lassa fever, and our own domestic experience of Rabbit Calicivirus.
Perhaps the authors could add to their ‘Prevention and Control’ section an admonition against research enthusiasm – in particular the ‘gain of function’ research which has been implicated in the origins of the Covid pandemic.