Advances in 3D printing technology could have profound implications for tissue engineering in plastic and reconstructive surgery.
3D printing has been a game changer across industries, including medicine (here, here, and here), yet its potential in plastic and reconstructive surgery remains largely unrealised. The primary issue lies in the limitations of current 3D printing technologies, which struggle to produce biocompatible materials at a practical speed and resolution.
Most 3D printers force a trade-off between speed and accuracy — high resolution prints take time, while faster methods lack precision. Additionally, many commonly used printing materials require extensive post-processing before they can be safely used in the human body.
For plastic and reconstructive surgeons, the ideal scenario is one where we can create and implant patient-specific, biocompatible tissue replacements directly in the operating theatre such as bone and cartilage or ultimately more complex composite structures such as organs. Given the fundamental principle of plastic surgery — to replace like with like — this remains a significant challenge. While skin grafts and synthetic implants have been useful, replicating complex tissues, such as bones and organs, has remained beyond our grasp.
In theory, this is where 3D printing should offer solutions, but existing technologies have failed to deliver on their promise due to fundamental limitations in printing speed, resolution and material compatibility.
The promise of dynamic interface printing
In collaboration with the Collins Lab at the University of Melbourne, we have explored dynamic interface printing (DIP) as a breakthrough technology in bioprinting. DIP uses light to cure or solidify photosensitive materials rapidly, producing high resolution 3D structures within a sterile environment — an essential requirement for medical applications.
Our method involves projecting images through a hollow tube into a bubble, where light solidifies a liquid resin or hydrogel in thin layers. By continuously updating the projection while moving the print head vertically, we can build complex 3D structures in a matter of seconds.
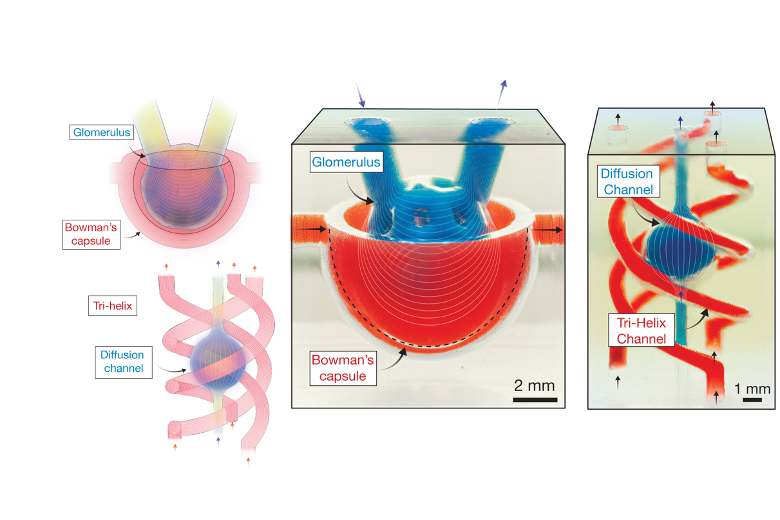
To overcome one of the biggest limitations of traditional 3D printing — slow material refill between layers — we introduced acoustic vibration to accelerate the process. This innovation, developed by David Collins and his PhD students Callum Vidler and Michael Halwes, allows us to create intricate structures at unprecedented speeds, with resolutions dictated by optics rather than mechanical limitations. Theoretically, we can achieve resolutions down to one micron, which is significantly smaller than most cell structures.
This has profound implications for tissue engineering. Unlike conventional bioprinting techniques, which struggle with producing structures with cellular precision, our approach enables us to print cell-laden hydrogel structures at a rate unmatched by any existing technology. Additionally, by manipulating acoustic frequencies, we can position cells during the printing process itself, aligning them in biologically relevant patterns — a crucial factor in tissue function.
Addressing vascularisation: a major breakthrough
One of the greatest hurdles in tissue engineering has been vascularisation. Once a printed tissue exceeds a millimetre in thickness, diffusion alone is insufficient for delivering nutrients, leading to cell death. This is why previous bioprinting methods have struggled to create functional human-scale tissues.
Most traditional techniques lack the resolution to replicate capillary networks, with extrusion bioprinting typically limited to structures around one millimetre in width. While some optical techniques can theoretically print at the scale of micro-vasculature, their slow print speeds compromise cell viability. Our DIP approach, on the other hand, provides a combination of speed and precision that could finally allow us to replicate the intricate vascular systems found in organs such as the liver and kidneys.
We have already demonstrated that our approach is highly biocompatible by printing a kidney-shaped model embedded with live kidney cells, which maintained high viability over several days. Beyond surgical applications, we are also exploring the use of our technology in disease modelling and drug discovery. By creating realistic tissue models, we can improve preclinical drug testing, reducing reliance on animal models and expediting the development of new treatments. Since our printer can generate hundreds of these models in a matter of minutes, this could significantly accelerate drug screening and personalised medicine research.
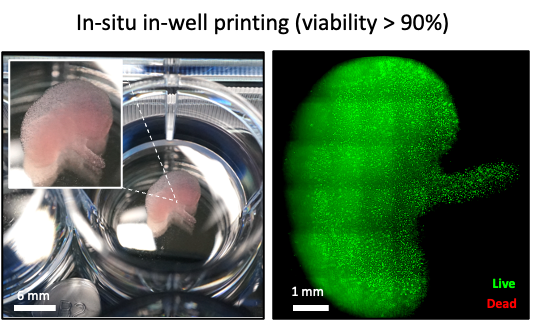
What comes next?
The potential of DIP is enormous, but to truly harness its benefits, we need strategic investment and policy support from the government and health institutions. Although we are in the process of commercialising this technology, widespread clinical adoption will require significant infrastructure changes within hospitals and research facilities.
One immediate priority is integrating bioprinting technology into hospital operating theatres. Having an in-theatre, user-friendly printer capable of producing customised tissue implants on demand would be revolutionary. However, this requires regulatory frameworks that balance safety with innovation, ensuring new techniques can be trialled and implemented without excessive bureaucratic delays.
Another critical area for government involvement is funding for translational research. While Australia has a strong research culture, there is often a gap between laboratory-based discoveries and clinical applications. Grants and incentives specifically for bioprinting could accelerate this transition, bringing cutting-edge regenerative medicine to patients faster.
From a broader health care perspective, bioprinting aligns with the shift toward personalised medicine. The ability to print patient-specific tissues using their own cells could reduce complications associated with graft rejection and improve surgical outcomes. In the long term, this could have positive implications to lower health care costs by reducing the need for organ transplantation and prosthetic replacements.
As a plastic surgeon, I hope for a future where bioprinting allows us to replace missing or diseased tissues with fully functional, patient-specific alternatives. While we are still in the very early stages, our work demonstrating DIP brings us closer to achieving this goal. With continued investment, collaboration, and regulatory support, we could witness a paradigm shift in reconstructive surgery — one where complex tissue loss is no longer a huge challenge, but a solvable problem with the push of a button.
Anand Ramakrishnan is the Head of Unit, Department of Plastic and Reconstructive Surgery, The Royal Melbourne Hospital.
Supported by: David Collins, Researcher; Callum Vidler, Researcher; Emmanuelle Koehl, Researcher; Michael Halwes, Researcher
Disclaimer: Authors Callum Vidler, Michael Halwes, and David Collins have co-founded a company involved in the development and commercialisation of the dynamic interface 3D printing technology described in this article. This affiliation is disclosed in the interest of transparency and does not affect the integrity or objectivity of the scientific discussion presented.
The statements or opinions expressed in this article reflect the views of the authors and do not necessarily represent the official policy of the AMA, the MJA or InSight+ unless so stated.
Subscribe to the free InSight+ weekly newsletter here. It is available to all readers, not just registered medical practitioners.
If you would like to submit an article for consideration, send a Word version to mjainsight-editor@ampco.com.au.